The Layman’s Guide to Electricity 2A: Generation Basics
Too much to cover in generation, so I broke it in half.
The Layman’s Guide series is a series of short articles going over particular aspects and principles of fields normally considered complicated or too academic for the average person. Emphasizing the principles and ideas that an average person may encounter in their daily life, while tying them together to the discipline as a whole, The Layman’s Guides are intended to be both a reference and an introduction. It is in essence what I wish I had when I was younger, a series of articles explaining key principles of a profession that allow one to begin exploring the field more holistically. This is the second part of the Layman’s Guide to electricity, meant to be a quick primer on how we make electricity at a grid scale, why we do it the way we do, the different ways to do it, their advantages and disadvantages to each way of doing so.
If you missed it, the first part is available below. If you want to know where on the electric bill we’re at, see the image below the link and above the divider.
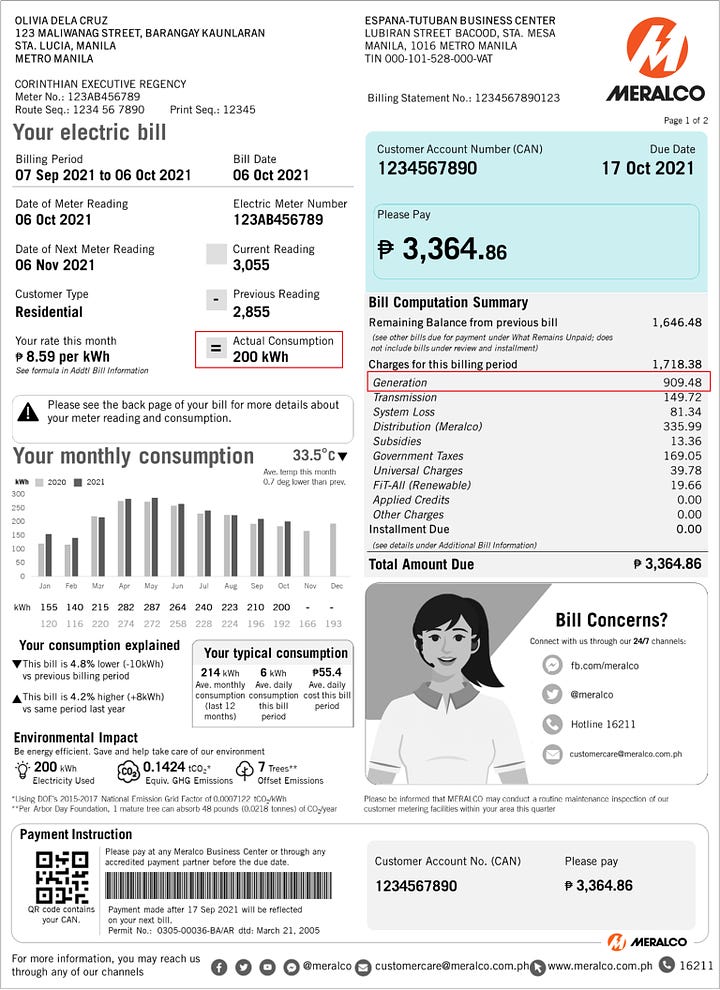
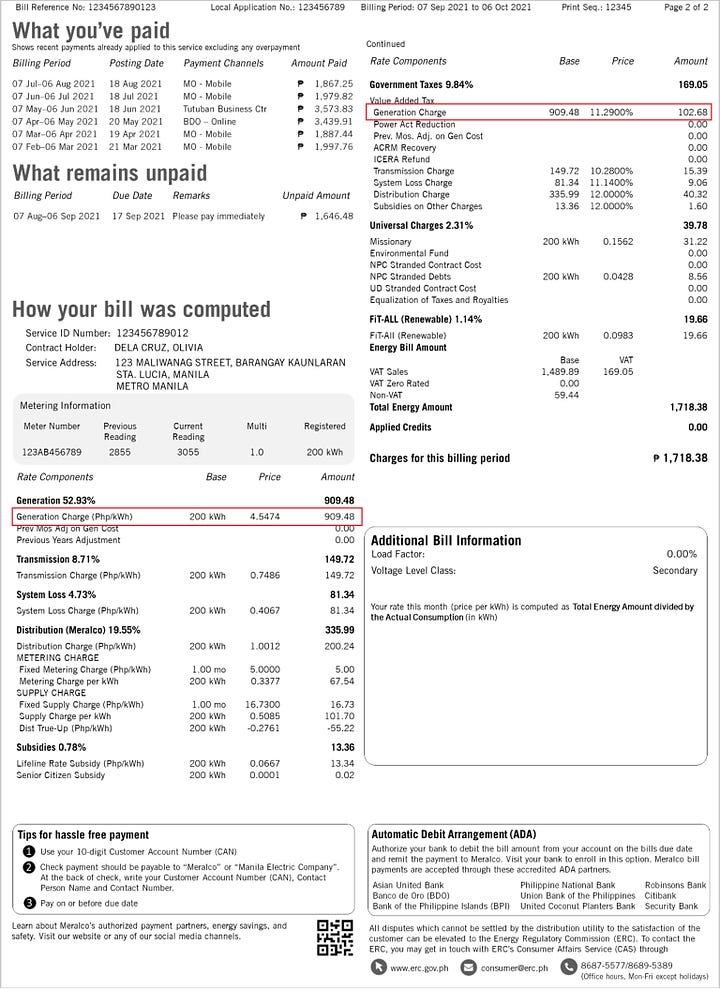
Volts, Amps, and Ohms: Watt you need to know, and why it Hertz
First, we’ll be talking about some of the different quantities you’re likely to encounter, each referring to a different attribute of electricity. Unlike what you’ll find in electrical books, however, I’ll be grouping and defining them by relevance/relation to the layman, rather than by physical principle. I’m going to group Volts, Amperes, and Watts together as the “power” group, with Ohms and Hertz being explained individually. That way, you have a better idea of their relationship when you look up more normal sources, which group together Volts, Amperes, and Ohms because of their physical relationship.
The Power Group – Volts, Amps, and Watts
Analogies between the two utilities, water service and electricity, are quite common, and will be used here for greater clarity. This resemblance is widely noted, but if you want a reference to help keep track, use the one in this footnote[1].
Volts (V)[2] – The unit of electric potential/electric potential difference. Best understood as “how much the power wants to go from one place to another”. Most analogous to water pressure.
Amps (A)[3] – Measure of electric current equal to one Coulomb per second. Best understood as “how fast the power is flowing from one place to another”. Most analogous to flow rate, or the amount of water flowing through the pipe or meter per second.
Watts (W)[4] – Equal to Volts x Amps (VA) in theory. Measure of the amount of electrical work is done by an appliance. Most analogous to the productivity of a water-powered grain mill – what you actually did with the electricity or water delivered.
Volts, Amps, and Watts together describe how much electrical power is being generated in total, how much is then delivered, and how much is wasted in the process. The technical terms here are apparent power (usually measured in Volt-Amperes), active power (usually measured in Watts), and reactive power (measured in Volt-Amperes Reactive to distinguish from apparent power). Apparent power is how much power is generated or taken into a system, while active power measures how much is applied by the appliance. In a perfect world, Apparent Power = Active Power, but there are always inefficiencies, ranging from the generation of heat, sound, and other by-products by the appliance, or electrical resistance of the system, which we’ll cover later. Reactive Power is that difference – referring to the power lost due to alternating current, magnetic intereference, line defects, so on and so forth.[5]
Electricians love using water and plumbing as an analogy, so I will follow in that grand tradition. Apparent Power is the pressure and flow rate water that comes out of the tank or reservoir. Reactive power is all the leaks between your reservoir and your swimming pool. Active Power is the pressure and flow rate at the swimming pool inlet.
The proportion of active power to apparent power is called the power factor – a power factor of 1.0 means a perfectly efficient system, while a power factor of 0 is a complete waste of energy. You can think of it as a measure of efficiency of a particular system.
Going back to the electric bill, the layman will only generally run up against Kilowatt-hours (kWh). These are measurements of Active Power multiplied by how long that power was delivered for (measured in hours). For a simple example, let’s take an LED Light bulb.
This light bulb uses 7 Watts of electricity to light up - so as long as you’re providing Watts, the bulb lights up. The number of hours in the month that that bulb is used will determine the number of Watt-hours that it contributes to your consumption for the period. In this case, keeping one of these bulbs open for an hour uses 7 Watt-hours of electricity. The basis of billing is the kilowatt-hour, equivalent to 1,000 Watt-hours. Do this for every appliance in your house, and you can estimate your own electrical use. This is particularly important if you’re sizing battery backup, solar generation, or generative capacity.
Anyone who travels overseas or imports appliances will likely have heard about voltage differences. Different countries standardize their grids on different voltages and frequencies, and the differences can have absolutely shocking results. Frequency differences are not so severe – as we’ll see later, 50 Hertz and 60 Hertz tends to only change the speed at which your appliance runs – but using the wrong voltage can have dramatic effects.
Years ago, my parents imported a Japanese massage chair. Coming straight from Japan, this chair was still designed for the 100 volt single-phase power they have there. While the single-phase standard power where I live is 220 volts, some outlets in the house are wired for 110 volts – close enough not to cause any problems, and easier to reach for local electricians. You can probably already tell where this is going.
At one point, my mom moved the chair and plugged the chair into a 220 volt outlet instead. The result was a loud pop as the electronics within fizzled out from having to deal with literally double the electrical force that they were designed for, breaking the chair’s electrical massage motors for good. Thankfully, my mother was unharmed from the experience, other than being the butt of the joke whenever it’s brought up.
For readers who are more familiar with water, imagine plugging a garden hose into a fire hydrant, or trying to shower with a pressure washer. You get the idea.
Some of the more rural, affluent, or preparedness-minded readers may have a diesel generator at their home or business. You’ll be happy to know that your generator is now an education tool – I’ll be using the nameplate on your generator as a learning aid. Here’s one as a sample.
Here we see a lot of how what we’ve talked about plays out. When you turn on the diesel motor, it spins and generates 100 kilovolt-amperes (100 KVA) in apparent power. It has a power factor of 0.8, producing 20 kilovolt-amperes reactive (20 KVAR) of apparent power, and 80 kilowatts of active power (80 KW). The power comes out at 230 volts (400 if all three phases are connected) at a frequency of 50 Hertz, up to a current of 144 amperes.
The limits on highest possible altitude and maximum ambient temperature are important because of the diesel engine. At too high an altitude, the engine begins to have a hard time pulling air and combusting it, while an excessively high ambient temperature will make the engine overheat.
Something important to note is that the copper lines coming to and from a generator must be sized to its maximum nameplate capacity. This will be important later on in the Guide, particularly when we talk about particular issues in electricity.
Now that we’re all caught up on how the power gets to you, let’s talk about frequency, and why it’s so important.
Hertz, frequency, and why it’s so important.
Hertz (Hz)[6] – Measures the frequency of an event and defined as one event or cycle per second. The clock’s second hand moves at a frequency of one Hertz. My fingers drumming on the tabletop impatiently waiting for an Internet page to load or kids playing with a light switch turning them off and on as fast as they can have an annoying frequency of about 2-3 Hz. May also refer to a rental car service[7].
Hertz and the frequency of the power grid are something somewhat uncommon for the layman to hear about but very important to the proper functioning of a power system. Hertz are a product of our use of Alternating Current (AC) rather than Direct Current (DC). To radically oversimplify, AC flicks back and forth between on and off at a very high frequency, while DC is simply powered on all the time. The former has many advantages in safety and transmissibility and so became the standard for most applications. Dare I say, AC performs dirty deeds, and DC stands for dirt cheap[8].
For readers who need a quick explanation – AC electricity isn’t actually always on, but always flipping between full on (+240) and full off (-240), a number of times a second equal to the frequency (Hz) of the current. See the photo below for a graphical explanation.
Ohms and the concept of electrical resistance
Ohms[9] (Ω[10]) – Measures electrical resistance between two points of a conductor based on Volts and Amperes. Defined as Volts over Amperes (V divided by A). Also used as a measure for electrical impedance, or the built-in resistance to alternating between the top and bottom of alternating current. In a water system, most analogous to friction and resistance from the pipes, as well as any machinery and pumps that use the system.
Electrical resistance – The opposition of a material to the flow of electric current. The reciprocal/opposite of electrical conductivity. Insulators such as rubber and most plastics fall into this category, as opposed to conductive metals, most commonly copper. Affects both AC and DC. One of the causes of reactive power.
Electrical impedance – The opposition of a material to the switching of alternating current. A complement to electrical resistance in causing reactive power.
In any physical system, resistance is inevitable. Nothing is perfect for any task, and we have to make do by using materials that are as available and as close to perfect as we can get. Ohms, electrical resistance, and electrical impedance embody this concept for electricity. All materials have a natural resistance to having an electric current pushed through them (electric resistance) and then have a resistance to moving between the peaks and troughs of alternating current (electric impedance). Spoiling a bit from the Transmission parts of the Layman’s Guide, this is one of the causes of system loss and will be important later when we talk about how lines are.
Resistors have a lot of uses in electrical work. They allow you to split voltages and currents, creating smaller circuits for personal use. Electric resistance also powers electric heating[11] – passing electricity through a conductor triggers its natural electrical resistance, causing the conductor to emit heat[12].
The Ideal Generator
Now that we’ve covered the fundamentals of electricity, we can put them all together in a basic description of a generator, starting from an illustration of how electrical power is generated. Note that this illustration is not meant to represent any individual system, but rather serve as an “ideal type” so that you can imagine everything else going forward. Readers that drive or work with cars will see a lot of similarities to how cars work, which I will expand upon as I go.
The Ideal Generator Model
Generally, most grid-scale electrical generators (and plenty of smaller ones) can be illustrated with the use of an alternator and an engine. An alternator uses a central spinning magnet (usually itself powered by electricity rather than being a permanent magnet) and coiled wires in order to generate electricity through the manipulation of electric fields – in short, the spinning magnet excites coils of wire and creates electricity. The magnet is spun with power from the engine, which in turn needs to be powered by a particular source – water evaporated into steam by coal, natural gas, nuclear reactions, or the movement of wind or water running a turbine or diesel pistons turning a crankshaft being the most common sources of spin[13]. Readers with more knowledge of electricity may point out photovoltaics as an alternative - don’t worry, we’ll get to those later.
This is an alternator[14], which car guys may recognize as the way an internal combustion engine car generates electricity. The engine spins the magnet (called the field winding or field magnet), which repeatedly flips the magnetic field in the coiled wires (the armature), generating an electrical current due to the repeated push and pull on the electrons in the wires. Alternators used at the grid scale create three-phase power using three pairs of coiled wires around a magnet, with each one of these phases corresponding to the single phase power most of us will familiar with. As an aside, three-phase is generated because machines used in industry and factories require a higher voltage because of their larger size and heating requirements, and customers can request three-phase power if needed. If you see a bank of transformers outside a facility that looks like the picture below, the place likely has three-phase power.
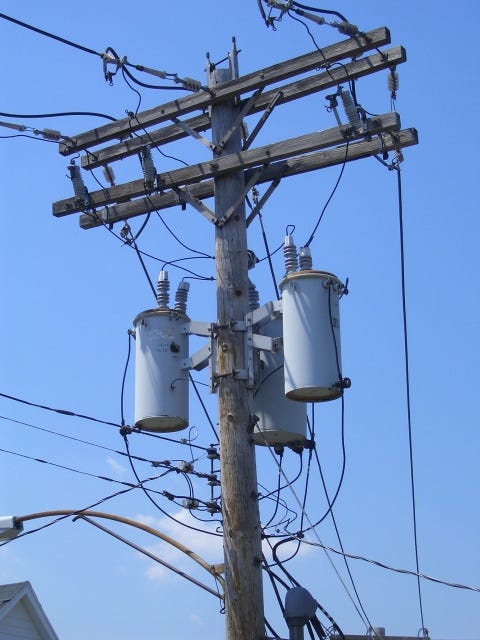
Moving on, alternators generate current based on the spinning of the magnet creating an electric field, which excite the wires. The frequency is controlled by the spin speed of the magnet, while the voltage is controlled by the strength of the magnet. So, if as the alternator above, there are six coils in the armature mounted around the magnet, each supplying one phase of power at 60 Hertz, you would need 60 rotations per second, or 3,600 revolutions per minute, to generate three-phase power for the grid.
The more interesting part of the alternator is the magnet. The magnet itself could be a permanent magnet, but the size required in power stations makes that impractical, so coiled wire with electricity running through it is used instead to control the strength of the magnetic field[15]. Yes, you heard that right, you need electrical current to make electrical current, usually provided by auxiliary or backup generators on site as I alluded to in part 1 when talking about black starts[16]. You can imagine that any interruption or fault in the power supply to the magnet coil could throw off the whole alternator, forcing it to shut down. If there is not enough spare capacity online, that one generator going off could disrupt the whole grid.
For that matter, grid consistency matters a lot. Think back to our Ideal Generator, where the strength of the magnetic field is controlled by the electricity supplied to the magnet coil. Should the grid frequency or voltage change, the magnetic field could change, which could itself cause the output frequency and voltage of the generator to change, triggering other generators, and so on and so forth until the voltage and frequency are out of control.
There are plenty of devices and procedures to prevent a runaway failure like this from occurring, but these are the kinds of risks the grid has to deal with. In accordance with this, they act quite conservatively, because one wrong move could send the whole thing tumbling down.
The idea that electricity requires the whole electrical system to function is going to come up often. Frequency and voltage are kept, as much as possible, within narrow bands in order to avoid damage to equipment and from faults cascading. The spin frequency and magnet strength of our ideal generator must be kept perfectly controlled in order to maintain the output, making it a very fragile system – which our electrical system is at its core[17]. I’m going to codify this as the Laws of the Grid, starting with the Law of Specificity.
The Law of Specificity: The electrical grid is meant to function within very narrow bands of performance. Careful management is required to make sure that abnormalities in generation, transmission, and distribution are smoothed out.
And a corollary, The Rule of Routine:
The Rule of Routine: The electrical grid likes to keep to established routines, large failsafes, and prefers reliable resources due to its narrow margins for error. Historically, the electrical grid was predominated by long-term contracts, representing the great importance of routine reliability to the industry.
The Photovoltaic Collector
Our ideal generator doesn’t cover how photovoltaic (PV) solar panels work, because in many ways, PV panels are the exact opposite of what we want from a grid-scale generator. The photovoltaic effect[18] involves sunlight causing the electrons in a material to be excited and be ejected from a material, taking their charge with them. Solar panels use semiconductors[19] like silicon or other metal alloys in thin wafers or films to “catch” these electrons and take their energy. They eventually return to the other side via diffusion, as the balance of electrons in the cell needs to be restored.
For a relatable example, think of a beach vacation. As winter approaches, and people living in colder climates dream of the hot sand and sun (excitation), there is a mass movement of people (electrons) from colder areas to warmer areas, where they spend a lot of money (electron charge) on accommodations, luxuries, and other stuff, before going back home when it’s less unbearably cold (diffusion). This GIF probably explains better than I can:
This is in many ways antithetical to the way the Ideal Generator, meant to represent most other grid power, works. The PV method produces Direct Current (DC), which has to be run through an inverter to be used as AC. Voltage is limited by how many cells you can connect in series in a single panel, as each cell only generates a very small amount of energy, leading to a low voltage. The constant trickle of electrons going back and forth creates sustained power with relatively low voltage as long as the sun is hitting the panel, giving you much less control over how much is made and when (contrast praying that clouds don’t block the panel with the ability to control the rotational speed and magnet strength in the alternator). A system that is tightly controlled within careful tolerances must play ball with this new, far less controlled, contender – not an active generator of electricity, in my opinion, but a passive collector of energy.
The Simultaneous Electrification Rule
I want to emphasize that I’m not talking about the reliability of the energy sources per se, but their predictability. Electricity, as a rule, must be used as it is generated, for two reasons. First, electricity is the flow of electrons. It is the flow, not the electrons, that powers our electrical devices. Second, storing or damming this flow is difficult, requiring chemical batteries or a physical storage method such as a large hydroelectric dam. This large bank of stored energy always runs the risk of being let loose. Fires in energy-dense lithium-ion batteries[20] are on the rise as adoption increases, and keeping too much water behind dams can cause dam failures[21] - catastrophic events where all the potential energy of the water is released at once. Even during normal times, dams will discharge water from the reservoir if too much of it builds up from excessive rain or melting snow, in order to protect the structure from damage. Storing power comes with its own dangers, which grow exponentially with the size of the storage. It often only takes one failure to cause a full breach.
With current technology, therefore, I believe we have enough foundation to propose the Simultaneous Electrification Rule, which goes as follows:
Electricity must be generated as it is used and used as it is generated, due to the difficulty and expense of grid-scale storage.
Together with the Law of Specificity and the Rule of Routine, this paints the picture of an industry that is very careful, being forced to synchronize demand and supply with very narrow margins for error. As such, the industry thinks in generous tolerances and follows routines and procedures – driven more by process than by profit. This is only bolstered by the prohibitive cost of putting up electrical wires, substations, and generation, requiring years of feasibility and environmental studies followed by years of construction and decades of debt in order to get anything done.
A fitting attitude for one of the backbones of the modern world.
Dispatchable and Non-Dispatchable Sources [Generators vs. Collectors]
Now that we’ve covered the ideal generator and the photovoltaic generator, and figured out some rules for how to think about electricity, we come to the part you’ve probably been waiting for – when we start talking about the different kinds of power stations and what they mean for you, the reader.
Well, we’re getting there. First, I’ll need to talk about how these stations are categorized, because you’ll hear these categories being thrown around even if you don’t exactly know what they mean. First, and most important, is the distinction between dispatchable and non-dispatchable power.
Dispatchable power[22] – Sources of electricity that can be programmed on demand at the request of operators according to market needs. Our Ideal Generator, representing most conventional power sources, is one of these dispatchable sources.
Non-Dispatchable power – Sources of electricity that cannot be called upon on demand according to market needs. Solar energy, wind energy, and run-of-river hydro, which are not under our control, are among these non-dispatchable sources. In my opinion, more properly called ‘collection’ rather than ‘generation’.
The difference between dispatchable and non-dispatchable power is important because of the Simultaneous Electrification Rule – every time you turn on a light bulb or plug something into a switch at home, the power operator has to have generation already running to meet your needs. Dispatchable power also gives you the ability to maintain the grid voltage and frequency, which is crucial because electricity is used in the alternators used to generate electricity in the first place, causing changes in the electricity generated, which can in turn affect other generators, until suddenly the whole power system is out of whack.
Connecting non-dispatchable sources to the grid, by that measure, is also a risk. While there are general patterns over when and how much power is generated by these sources (solar, for example, when the sun is out, and wind when it’s blowing in an area with turbines), a key issue is that you’re never sure ahead of time of this fact. If a cloud unluckily happens to float over the solar farm, or the wind blows so fast they have to shut down the turbines to avoid damage[23], the expected electricity may not materialize, forcing dispatchable sources to pick up the slack. If you want to read about this more in detail, you can look up the “intermittency problem in electricity” – I’ve added some[24] footnotes[25] on[26] the[27] subject[28]. Frankly, it’s very contentious, and a lot depends on how battery technology develops and how willing we are to bet against “freak” accidents like the 2021 Texas power outage[29] caused by a blast of extreme cold that did all of the following:
Suddenly increased demand by forcing Texas homes’ heat pumps to switch to electrical resistive heating, creating huge demands.
Reduced solar generation via storm clouds.
Froze the wind turbines solid, because ice throws off the balance of the blades and can cause damage to the turbine if run in those conditions.
Froze the natural gas pumps, because the electrically-powered gas pumps had their connection to grid disrupted by the sudden withdrawal of wind electricity.
Forced ERCOT to start “load shedding”, or basically disconnecting somepeople, to preserve voltage and frequency in light of these disconnections to prevent the whole grid going down.
More qualified people than me can figure out who went wrong and why, but I’m a simple man – I would strongly prefer it if my power worked when it was cold and needed it most, thank you. To that end, I’m always in the corner of a good mix of dispatchable electricity, mixing a core of always-running plants, a few that react quicker to pick up the slack, and some just in case the worst happens. That’s also one other way to chop up the dispatchable generation, as we’ll see below.
Cheat sheet for Dispatchable vs. Non-Dispatchable:
Dispatchable - all conventional generation, conventional dammed hydro, pumped hydro storage.
Non-Dispatchable: Renewables.
Baseload, Mid-Range, and Peaking
Dispatchable generators are divided into three types, in order of how they are best used, each detailed below.
Baseload Plants[30] – Power plants that are best used to service the base load, which is general demand that lasts throughout the whole day. Usually uses whatever’s abundant in your area, with a preference towards older steam turbines running on cheaper fuels like coal, nuclear, or the very efficient combined-cycle natural gas. Ideally also the cheapest to run, because they’re always running.
Mid-range Plants[31] – Power plants that increase or decrease output to match variation in electricity throughout the day. Normally made up of smaller but faster sources such as fuel oil, diesel, geothermal, run of river, or combined-cycle natural gas. Coal and nuclear can also load-follow, but do not respond immediately. Usually more expensive than base load because not all of your capacity is being used at any one time.
Peaking Plants[32] – Power plants that are only activated in case of sudden surges in demand, usually the oldest generation in the fleet. Traditionally, this role was filled by fuel oil plants and diesel generators, but can also be filled by combined-cycle natural gas turbines. Replacing these is a use case for battery storage.
While Wikipedia also lists wind and solar with storage as potential base load or mid-range plants, I will exclude them for the time being pending the discussion on batteries (which I will postpone until, at the earliest, the next part of the Guide). We will focus for now on the naturally dispatchable sources of energy, since they are the majority of the existing generation fleet and will continue to be so for a long time coming.
Base load power is turned on all the time, if not to full capacity, and is used to handle the perennial demands of the electrical grid. In general, what ends up as base load where you are will be based on “whatever’s abundant”. Conventional dammed hydropower can handle base loads with a large enough reservoir and a small enough demand, or with abundant river valleys. The mainstays of this category, however, are coal and nuclear. These both share the cost characteristics of having a large upfront cost (the plant) with relatively cheap fuel (coal is cheap, and nuclear needs very little of the expensive fuel at a time) running a steam turbine. Steam turbines are slow to start but are easy to run once they’ve gotten going. To understand why, simply put some water in a pot and boil it with the lid on. At first, the water doesn’t want to heat up, but once it gets to a rolling boil, it keeps giving off steam even if you turn down the heat. Imagine that, but on a massive scale, and you can imagine it would take some time to get one of these started – time that might see the grid in trouble. In contrast, ramping production up and down, while slower, is still quicker than starting the plant. In order to make sure you can always turn it up, therefore, you never turn it off.
Mid-range power is also turned on all the time and not to full capacity, but it has a different purpose. In the United Kingdom, there is a phenomenon called TV Pickup[33], where people all over the UK use their electric tea kettles to brew themselves a cup during the commercial breaks of popular TV shows. This forces the grid authority to have mid-range and peaking plants on standby to service that demand, ramping up generation in order to prevent a drop of grid frequency and local voltage . Mid-range power is a balance between “cheap to run” and “quick to start”, best exemplified by the natural gas single-cycle plant. Natural gas is cheaper than fuel oil and diesel but more expensive than coal, but uses an internal combustion engine to make its power. This makes it quicker to respond than the steam turbine – closer to hitting the gas pedal in your car rather than waiting for water to boil, making it an ideal fuel for mid-range power. Combined-cycle gas turbines have the best of both worlds[34] – they burn natural gas in what is essentially a giant jet engine to make power, then they use the exhaust from the turbine to make steam to run a steam turbine. This grants them increased efficiency when run as base load, with the ability to start up quickly to run as a peaking plant.
Said peaking power is exemplified by two equal and opposed kinds of generation – diesel generators and pumped hydro storage. Diesel generators[35], unlike most of the types of generation we’ve discussed, are not limited to the grid, but go all the way down to being owned by individuals and small businesses that need reserve power, particularly fuel stations that use them to run the pumps when the power’s out, so you can buy fuel to run your own generator. Expensive to run because of the cost of the fuel, these generators have the advantages of being quick to start and readily available, being essentially car engines spinning oversized alternators – almost exactly like our Ideal Generator.
In contrast, pumped hydroelectric storage[36] is a rare investment available only to relatively large users and utilities. The concept is pretty simple: When electricity is cheap or abundant because of slack demand, you pump water up above the dam into the reservoir. When electricity is expensive, you open the floodgate to the mechanical turbine, spinning it to generate electricity and quickly balance the load. This instant release allows the pumped hydro plant to respond quickly to emergencies, such as the aforementioned TV Pickup. While this is an oversimplification of the business strategy of pumped hydro storage, the idea holds in general.
Peaking power, however, is necessarily expensive. Diesel generators (in aggregate) and pumped hydroelectric storage (dams) are expensive, and these costs need to be paid off over the short periods of time that they are generating power. As such, they will charge a lot for these services, to cover the costs of holding them in reserve for when the time comes.
In summary:
Baseload wants to be running all the time – cheap power that’s hard to start up and slower to adjust.
Mid-range wants to be running all the time – slightly more expensive power that’s quick to adjust when needed.
Peaking generation ideally never runs – it’s in reserve when you need it, but it’s very expensive.
When we get to working backwards from the bill, this will be very important, but for now, file it away. It’s important to remember that though all plants will fall into one of these three categories, and it is possible to guess based on the fuel what the output will be, it often depends heavily on availability, grid size, and plenty of other complex concerns that more qualified people can handle. For now, this cheat sheet will suffice:
Base load: Conventional hydro power when abundant, steam turbines (coal, nuclear, traditional natural gas), combined-cycle natural gas.
Mid-range: Geothermal, combined-cycle natural gas, run-of-river hydroelectric. Newer coal and nuclear can also fill this role.
Peaking: Diesel or fuel oil combustion, pumped hydroelectric storage, turbine only combined-cycle natural gas.
Conclusion
We’re only half done and haven’t even gotten to a discussion of each individual source of generation yet, or a quick discussion of batteries. As such, I am going to split our discussion on generation into two parts.
Coming Up: Part 2B: Generation by Type of Power
Yep, that’s right, there’s more. In a terrible tragedy, this piece has blown out long enough that I feel it would be better to cover the different types of power used for generating electricity in the next part. Oh no, more Layman’s Guide. Bear with me, dear reader, because I think it’s for the better.
Feel free to use the comments section to offer insights, corrections, or just talk about electricity. I love this stuff, so if there’s anything you thought could use more detail, I’ll be happy to respond! Long-form comments can be directed to my e-mail at argomeditations@proton.me. If I get a particularly insightful conversation, I’ll publish it for everyone’s edification.
Thank you for making it to the end! If you’d like to support the Layman’s Guide, like, share, and leave a comment. If you really want to support my Guide, use Paypal to buy me a coffee at this link - this is also where I’ll be putting out a collected and revised edition of the Guide as a PDF when it’s all done. Please don’t do a monthly donation, by the way - I can’t guarantee I’ll have a monthly product for you.
I would enable paid Substack instead, but Stripe doesn’t operate in my country yet, so.
[1] https://www.e-education.psu.edu/ae868/sites/www.e-education.psu.edu.ae868/files/Optional_Review/Electricity_An_Analogy_HP87.pdf
[2] https://en.wikipedia.org/wiki/Volt
[3] https://en.wikipedia.org/wiki/Ampere
[4] https://en.wikipedia.org/wiki/Watt
[5] https://control.com/technical-articles/active-power-reactive-power-apparent-power-and-the-role-of-power-factor/
[6] https://en.wikipedia.org/wiki/Hertz
[7] https://www.hertz.com/rentacar/reservation/
[9] https://en.wikipedia.org/wiki/Ohm
[10] Alt+234 on Windows, Option+Z on Mac.
[11] https://en.wikipedia.org/wiki/Joule_heating
[12] Note that you do still need to use a conductor for this, otherwise the current won’t travel through. A material with too high of a resistance will simply fail to let the current go through, meaning you can’t heat your house more efficiently by electrocuting rubber. Very sad, I know.
[13] This is the same concept – diesel generators use crankshafts to turn the alternator the same way your car uses the crankshaft to turn the wheels (indirectly). Car guys probably screaming at me right now.
[14] https://control.com/textbook/ac-electricity/polyphase-ac-power/
[15] For those wondering, you can’t calculate amperage until you have a load, so at the generation facility proper, there’s no way to control the amps. That comes later, we’re still a long way from the meter.
[16] https://en.wikipedia.org/wiki/Black_start
[17] Real generating plants have plenty of failsafes to deal with this sort of thing. Just know that a lot of work and money goes into keeping things running.
[18] https://en.wikipedia.org/wiki/Photovoltaic_effect
[19] https://en.wikipedia.org/wiki/Solar_cell#Materials
[20] https://www.nfpa.org/education-and-research/home-fire-safety/lithium-ion-batteries
[21] https://en.wikipedia.org/wiki/Dam_failure
[22] https://en.wikipedia.org/wiki/Dispatchable_generation
[23] https://www.endesa.com/en/the-e-face/energy-sector/wind-turbines-stopped-with-wind
[24] https://blogs.scientificamerican.com/plugged-in/renewable-energy-intermittency-explained-challenges-solutions-and-opportunities/
[25] https://www.powermag.com/solving-the-intermittency-problem-with-battery-storage/
[26] https://www.raycandersonfoundation.org/articles/solving-the-intermittency-challenge-the-importance-of-grid-storage-as-renewable-electricity-rises
[27] https://www.manhattancontrarian.com/the-disastrous-economics-of-trying-to-power
[28] http://euanmearns.com/el-hierro-fourth-quarter-2018-performance-update/
[29] https://en.wikipedia.org/wiki/2021_Texas_power_crisis
[30] https://en.wikipedia.org/wiki/Base_load
[31] https://en.wikipedia.org/wiki/Load-following_power_plant
[32] https://en.wikipedia.org/wiki/Peaking_power_plant
[33] https://en.wikipedia.org/wiki/TV_pickup
[34] https://en.wikipedia.org/wiki/Combined_cycle_power_plant
[35] https://en.wikipedia.org/wiki/Diesel_generator
[36] https://en.wikipedia.org/wiki/Pumped-storage_hydroelectricity
Good summary of how electricity works and the concept of reactive power, which is when the voltage and current are out of sink leading to the power factor. In single phase power is P=VI (voltage times current) but in three phase its (Square root 3)x VxI Cos(phase angle) which is the phase angle shift between current and voltage, on most UK motors that's 0.9.
Well done for its intended purpose. As a former professor of science and engineering topics, I see a few *minor* items that could be corrected. I look forward to further installments.