The Layman’s Guide series is a series of short articles going over particular aspects and principles of fields normally considered complicated or too academic for the average person. Emphasizing the principles and ideas that an average person may encounter in their daily life, while tying them together to the discipline as a whole, The Layman’s Guides are intended to be both a reference and an introduction. It is in essence what I wish I had when I was younger, a series of articles explaining key principles of a profession that allow one to begin exploring the field more holistically. This is the second part of the second part of the Layman’s Guide to electricity, meant to be a discussion of the different ways we make electricity at the grid scale and a discussion of their relative merits based on current deployed technology as of the time of this writing.
The previous parts of the Guide are available below. If you want to know where on the electric bill we’re at, see the image below the link and above the divider. You will need the second link to fully understand this link, particularly the last section on dispatchable vs. non-dispatchable power and the discussion on base load, mid-range, and peaking power plants.
https://argomend.substack.com/p/the-laymans-guide-to-electricity-bde
If you want to support The Professional Amateur and get Layman’s Guides out faster, follow the Ko-Fi link in the button below. These Guides take a lot of time to research, write, and put together, so if you like them, please support me in making them happen. Leave your Substack handle as a message to me, and I’ll be including you in the sponsor roll on the next part - members get their own section!
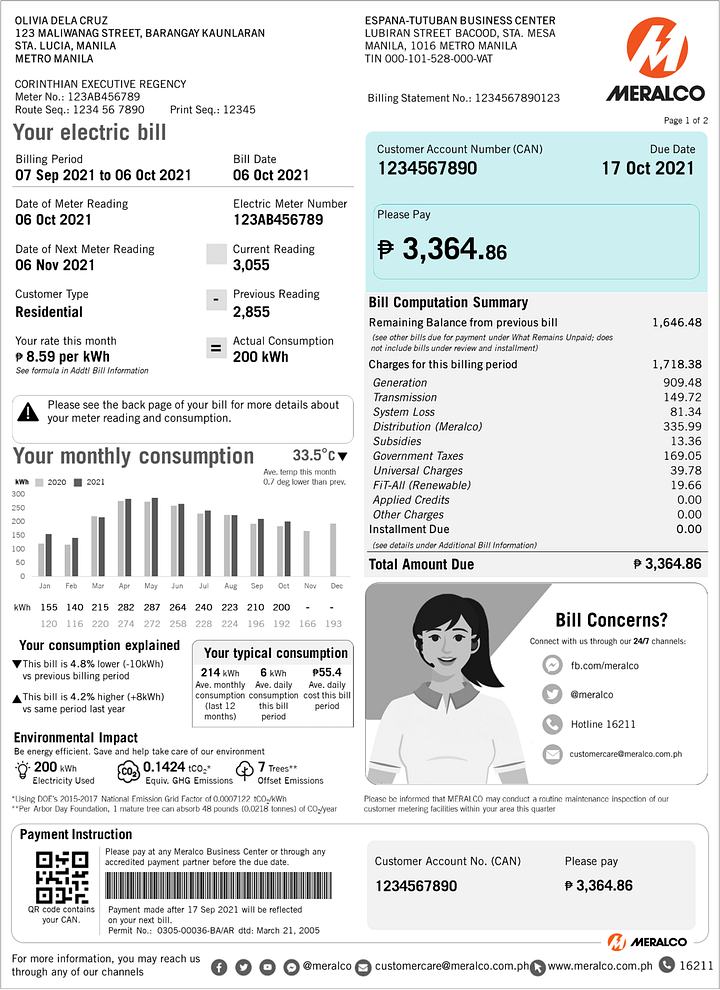
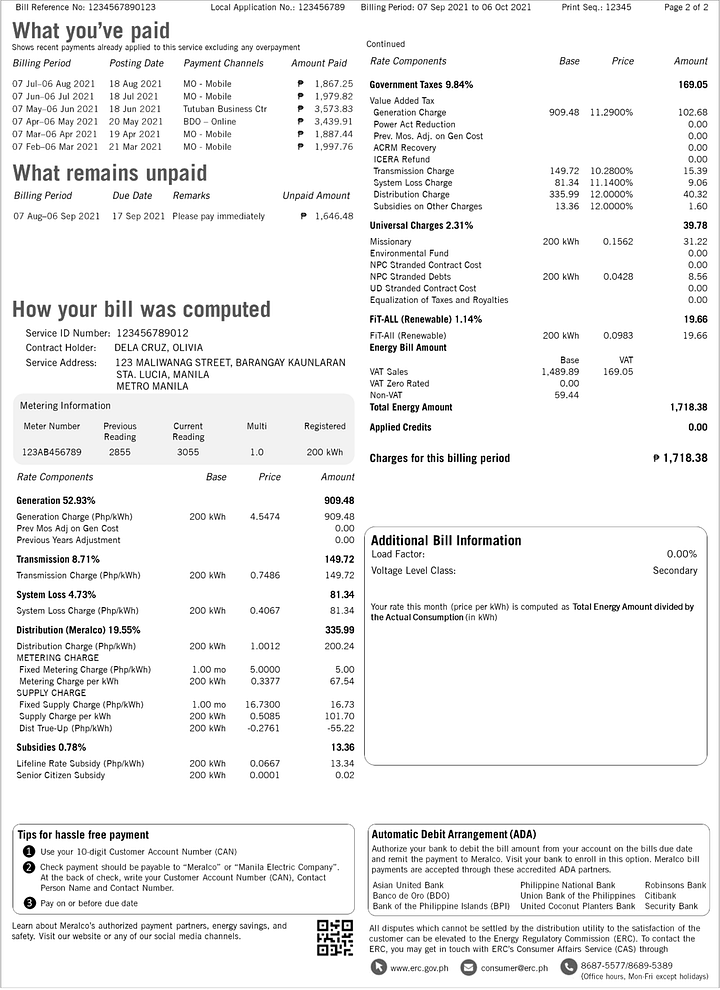
The Power Principles
If you’re reading this without reading the previous part of the Layman’s Guide, I’m going to reiterate the Power Principles and their cheat sheets here.
The Law of Specificity
Every part of the electrical grid is meant to function within very narrow bands of performance. Careful management is required to make sure that abnormalities in generation, transmission, and distribution are smoothed out.
Corollary - The Rule of Routine:
The electrical grid likes to keep to established routines, large failsafes, and prefers reliable resources due to its narrow margins for error. Historically, the electrical grid was predominated by long-term contracts, representing the great importance of routine reliability to the industry.
Simultaneous Electrification Rule
At the grid scale, where storage is not viable, electricity must be created at the same time as it is used, unless stored via pumped hydro or the newer battery storage.
Dispatchable vs. Non-Dispatchable
The distinction of dispatchable vs. non-dispatchable refers to whether you have control of when you generate or when you don’t.
Dispatchable - all conventional generation, conventional dammed hydro, pumped hydro storage.
Non-Dispatchable: Renewables, run-of-river hydro.
Plant Economics
Generating plants generally fall into one of three types; baseload, mid-merit, and peaking plants, based on their role. Baseload plants are run all the time to cover the base load, mid-merit kept running low and sped up to meet changes in load, and peakers used in emergencies to meet spikes in demand.
Base load: Conventional hydro power when abundant, steam turbines (coal, nuclear, traditional natural gas), combined-cycle natural gas.
Mid-range: Geothermal, combined-cycle natural gas, run-of-river hydroelectric. Newer coal and nuclear can also fill this role.
Peaking: Diesel or fuel oil combustion, pumped hydroelectric storage, turbine only combined-cycle natural gas.
Orientation
In this part of the guide, we’ll quickly discuss the different generator feedstocks in order of when they’re most important/significant, followed by a discussion on renewables (which I consider collectors, rather than generators), and batteries. We’ll also study how they fit in as non-dispatchable sources into a grid built around dispatchable generation, and the implications for policy.
Humans have done a lot of things to make energy, so I’m going to make this quick. I’ll be using this format to talk about each fuel.
Heading (Type of Fuel)
Policy Reaction:
Type: Generator (dispatchable) or Collector (non-dispatchable)
Produces: Electricity, Heat, and/or Mechanical Work
Specialty: Base load, Mid-Range, Peaking, Special, Small-Scale
Contribution: The percent of worldwide electricity generation attributed to this source, if reported separately, based on Our World in Data[1].
Details: A quick blurb on the power type.
Muscle Power
Policy Reaction: Very obsolete.
Type: N/A
Produces: Mechanical Work
Specialty: Special, Small-Scale
Contribution: N/A
Muscle power, most often provided by humans and animals, is the first and most prevalent mode of power. Starting with simple hard work like digging with your hands or punching trees in Minecraft, the use of muscle power was refined over eons. Treadwheel cranes[2] or horse mills[3] used to be economic methods of generating power for the difficult tasks of lifting stones and grinding grains. Ancient landmarks are built mostly on the back of this form of power.
In the modern day, the use of muscle power is reserved for tasks that can only be performed by the humans/animals concerned, due to its relative inefficiency compared to other methods. Rather than doing the work ourselves, we came up with all these other things to do it for us.
Biomass
Policy Reaction: Encouraged for sustainability, but limited potential.
Type: Generator
Produces: Heat, Electricity
Specialty: Peaking, Small-Scale
Contribution: 2.35% of global electricity.
The use of recently living (now dead) organisms for bioenergy production. Wood, straw, organic waste, and cultivated switchgrass or napier grass are common feedstocks[4], along with inorganic waste as part of waste-to-energy programs. Firewood also falls into this category, where the biomass is burned for its heat. Dry dung fuel[5] is also traditional in many countries for heating, with the downside of organic and inorganic pollution coming off the burning dung, particularly due to heavy metals in the dung oxidizing the body[6].
Today, biomass is best used as a small, dispatchable peaking source. The thermal efficiency of biomass is far lower than other hydrocarbons, making it difficult to generate the large amounts of electricity and heat that other sources allow. The fuel is also limited by the size of the land area available for growing it, making it difficult to scale up.
Water Power (Run-of-River)
Policy Reaction: Neutral and encouraged, but limited by geography and usefulness.
Type: Collector
Produces: Mechanical Work or Electricity
Specialty: Small-Scale
Contribution: Not reported separately from Reservoir Hydro
Run of river water power is probably the oldest form of renewable energy. Loggers in the old days, rather than carry the logs back to the mill, floated logs down the river[7] to sawmills, themselves powered by the run of the river, to be cut. These were likely powered partly by water wheels[8], themselves an ancient technology dating back to the Hellenistic Period, Rome, India, and China. They are the distant ancestor of turbines, which are built to withstand faster revolutions, and be more efficient.
Run of river water power works as a small version of the popular hydroelectric dam and reservoir system – only partially diverting the river, rather than stopping it and forming an artificial lake. A small dam is inserted to create a pond. At the bottom of the pond and going down to the electricity-generating turbine is the penstock, a narrow pipe through which water is pressurized to run the turbine, which generates electricity. After this is done, the water is released downstream[9].
Run-of-river’s main advantages are the low effect on the river, the relatively small investment required and the ability to scale down, providing power at a local scale. The natural rhythms of the river are not disrupted by run-of-river generation, unlike large reservoirs that flood a wide area. Far-flung communities or homesteading individuals definitely appreciate this ease of use and installation, making run-of-river a common small-scale choice.
Run-of-river’s main weaknesses are its intermittency and limited size. While it’s quite easy to find a river, finding one with sufficient flow to provide electricity year-round, or even reliably, is difficult. Without pondage functioning as a small reservoir, you are also at the mercy of the river’s flows from moment to moment. Scale up the reservoir too much, and you lose the benefits of cheapness and limited environmental impact. Run-of-river also does not help with flood control or water storage – both of which are also very good reasons to dam your rivers.
Water Power (Reservoir Hydropower and Pumped Hydro Storage)
Policy Reaction: Generally supported but highly limited by geography. Effects on wildlife are also a concern.
Type: Generator (dispatchable)
Produces: Electricity, Water Reserves
Specialty: Base load, Peaking
Contribution: 14.96% of global electricity production. This number includes run-of-river generation.
Conventional hydroelectric dams are a marvel of engineering, serving two purposes key to human survival and thriving. The key element is a large concrete wall (the dam) blocking a river and filling up its valley to make a large and deep lake (reservoir) that provides pressure for the water coming from the dam[10]. The dam has gated penstocks that allow the water into the turbine room to spin the turbine to generate electricity before allowing the water to follow the course of the river. By increasing water storage and generating electricity, hydropower plays a role in two of the most crucial modern utilities, electricity and water.
These dams can, and are often built to, serve multiple simultaneous purposes. First, the production of electricity as described. Second, the dam can store large amounts of water in the reservoir for use during the dry months in irrigation or as drinking water. Third, the dam can run the reservoir down in advance of heavy rains, allowing it to store water and control flooding along the river when needed. These three are cross-purposes, however, and the dam operator must balance these three carefully.
In terms of electrical generation, conventional hydro provides a very interesting resource. It is a baseload plant – but unlike the traditional base-load coal plant, the hydroelectric dam can ramp up from cold start to full operation within ten minutes[11], compared to steam turbines which take half to a full day, or combined-cycle turbines that take one to twelve hours.
This also directly contradicts the reservoir’s mandate to store water for irrigation or drinking, which is compromised by the early release of water. If you hold the water back, however, it also contradicts the reservoir’s other mandate of flood control, since the reservoir cannot hold more water than it is designed for. Doing so risks opening emergency spillways to allow water through the dam and causing unexpected floods downriver, or worse, a catastrophic dam failure that releases the contents of the reservoir in a single devastating flash flood.[12] The risks of silt deposits blocking the dam’s intakes or extended dry periods depleting the reservoir should also be considered – the power a dam produces is difficult to guarantee. The management of a hydroelectric dam is a constant balancing act of cross-purposes, underscoring the vital importance of both electricity and water to human society.
There are environmental costs to a hydroelectric dam as well.[13] While emitting less carbon dioxide than other base-load plants do to generate electricity, the environmental effects of a dam can be staggering. The planned reservoir area can include existing human settlements and wildlife – a river valley is prime real estate because of the source of fresh water, and likely already has a lot of wildlife living there. Indigenous peoples[14] and endangered species[15] are often threatened, particularly fish that use the river, like salmon.
Pumped storage provides a partial mitigation of some of these consequences, at greater expense and engineering complexity.[16] By adding a pump that pumps water from a downstream reservoir back up to a higher reservoir, pumped storage can take advantage of periods of cheap electricity to recharge the reservoir, allowing fuller use of the flexible power provided by hydroelectricity by “storing” the cheap electricity as water going uphill until needed.
As it stands right now, this is the best-known and current most practical method of grid-scale storage, and this dynamic is one we will revisit later when talking about batteries.
King Coal
Policy Reaction: Can’t live with him, can’t live without him.
Type: Generator (dispatchable) or Collector (non-dispatchable)
Produces: Electricity, Heat, and/or Mechanical Work
Specialty: Base load, Mid-Range, Peaking, Special, Individual
Contribution: 35.63% of global electricity production
Coal was the fuel for the steam engine, the beating heart of the Industrial Revolution and the fuel that powered the first railways[17]. It replaced the hottest-burning commercial fuel of the time, charcoal, in smelting iron, saving countless trees from being cut down for charcoal. Abundant, inexpensive, and distributed all over the world, coal-fired steam turbines are the base-load backbone of the world’s electricity supply. Most of the oldest generating facilities around the world run on coal, for the good reason that coal was the first global industrial-scale feedstock. There’s a lot for the casual student to catch up on – let me give you a quick rundown.
First, all coal is sorted into grades. These grades are based on the moisture content and dry carbon content of coal, with higher carbon content and lower moisture content being associated with greater heat content, making the fuel more efficient. The grades are lignite, sub-bituminous, bituminous, and anthracite, along with a grade lower than lignite called peat. Peat is the first step in the formation of coal – needing higher temperature and pressure to be turned into lignite. The more pressure the coal is subjected to, the finer the grade becomes, taking millions of years to form[18].
Regardless, any coal above then has to be dug up in mines and ground up to form a good feedstock. Coal arrives at plants by rail, ship, train, or slurry pipeline at the plant[19], all crushed into pieces less than 5 cm in size for ease of burning[20]. Once there, we can get to the different types of coal boilers. Fluidized beds use coal embedded in a hot, bubbling bed of ash and other particulates, jets of air providing the oxygen for the coal combustion that allows heat transfer and chemical reactions within the bed, as well as reducing pollutants[21]. There are even variants of the fluidized bed that combine cycles – converting coal to gas through pyrolysis, combusting that gas, and then using the exhaust to run the turbine, called circulating fluidized bed[22] and integrated coal gasification cycle technologies[23], though applications of these are limited due to the material requirements and engineering complexities involved.
Then we get to the steam. As technology has advanced, we have become more able to subject the steam in the turbine to ever-higher temperatures and pressures, allowing newer coal plants to run at greater efficiencies. The oldest plants are called subcritical, working with steam pressures below 3,200 pounds per square inch and temperatures below 1,025 degrees Fahrenheit (550 degrees Celsius). Below the critical point of water at 3,200 psi, the steam still behaves like a gas and delivers less power – providing about 34% efficiency. The next step up is supercritical, at 38.5% efficiency, where the boiler pressure is pushed to about 3530 psi, and temperatures up to 1,050 F (565 C). At this higher pressure, the water still acts like a fluid, imparting more force to the turbine and increasing efficiency to around 38%. The current bleeding edge is in ultra-supercritical coal plants, ramping pressure up to 5,300-5,600 psi and 1290-1330 F (700-720 C), possibly reaching 44-46% efficiency.[24] This constant improvement of coal may be about to be upstaged – General Electric Vernova boasts of an advanced ultra-supercritical coal plant that might deliver even greater efficiency, possibly reaching 50%.[25]
Coal is emblematic of the entire generation industry. It is the canonical base-load plant – slow to start up and warm up, but very cheap to run as long as it stays running. This is due to the requirement for high pressures and temperatures in the steam boiler – it takes time to get up to those temperatures and pressures, approximately one to three days depending on the specific plant[26]. Since those temperatures and pressures directly affect the speed at which the turbine turns, it is difficult to adjust or quickly add or reduce generation from the coal plant, making it so that steam turbines want to be run at full blast as much as possible, in order to maximize temperature and pressure. The endless search for greater temperatures and pressures – and thereby greater efficiencies – has led us to continue improving on the existing formula, pushing us into supercritical and ultra-supercritical coal. Concerns about emissions led to the fluidized bed reactor, which includes reagents that react with pollutants and bind them as the coal is burned, preventing the emissions right at the burner.
Providing the largest share of world electrical generation despite newer and more efficient methods popping up, coal is a proven and relatively cheap (in many places) source of generating capacity, and will likely be an important contributor for years to come. As humans continue to demand more and more electricity, King Coal will be there, ready to form the base-load upon which all others will stand.
Queen Oil
Policy Reaction: Burn less of it for electricity. She’s both too polluting and too versatile to keep doing it.
Type: Generator
Produces: Electricity, Heat, Transportation, industrial feedstock, need I go on?
Specialty: Peaking, Individual
Contribution: 3.16% of global electricity production.
Oil, first considered a waste product when discovered, is now one of the world’s most important commodities. This “black gold”[27], as it was once called, comes out of the ground as crude oil, each different well with its own unique mix of chemicals contained within the black sludge – generally similar but not so similar that the same techniques can be used. This is why the market for crude oil is actually three different benchmarks – Brent, West Texas Intermediate (WTI) and Dubai/Oman, each representing a different general crude chemistry. The most important factor is the sulfur content – sulfur is an impurity, and less sulfuric oils are called “sweet”, while more sulfuric ones are called “sour”[28]. This crude oil is then distilled to separate its components – many of which you will likely recognize in the image below. For our purposes, the most important members of this stack for our purposes are two of the lowest down – residual fuel oil or bunker fuel at the very bottom and distillates.
Residual fuel oil is the lowest grade of oil, and it is the grade most often used in power plants and seagoing vessels, being combusted to provide heat to run steam turbines or fuel for massive combustion engines – gigantic versions of the combustion engines used in cars. This oil is generally considered a waste product from refining the higher, more desirable grades of oil[29].
For the individual user running a diesel generator at home or for a business, the important category is distillates[30]. Distillates include fuel oils used for home heating, diesel fuel for automobiles and railroad locomotives, and the fuel stock for burners and trash incinerators. This crude is lighter, sweeter, easier to transport, and burns cleaner than residual fuel oil thanks to having less sulfur. This is a very important consideration because this fuel is burned closer to more people, making the greater release of pollutants from burning a lower grade of fuel unacceptable.
Oil is complicated. It deserves its own Layman’s Guide. Everything here does, really.
Where oil really shines is in its use in transportation. In the United States, for example, 27% of all total energy (which includes all electricity) is used for transportation. Of that 27%, 52% (14% of all energy) comes from gasoline, 22% from distillates (5.94% of all energy), and 12% (3.24% of all energy) from jet fuel, both of which are oil products[31] totaling 23.18% of total energy. Remember that all electricity is only 20% of all energy consumption worldwide, which, if we take the proportions here as representative, means that oil provides more of the world’s energy needs than electricity does. It is hard to overstate the importance of oil, and so I crown her Queen, though if we’re looking at total energy, she may be no mere Queen, but the Empress.
Natural Gas (Combustion and Combined-Cycle Gas Turbine)
Policy Reaction: The best of the polluting bunch.
Type: Generator
Produces: Electricity, Heat
Specialty: Base load, Mid-Range, Peaking – a wonderfully flexible fuel!
Contribution: 22.48% of global electricity production.
Natural Gas is a gaseous mixture of hydrocarbons made predominantly of methane. Originally a by-product of oil production, natural gas is a light vapor that comes off of crude oil when the pressure is reduced by drilling into the reservoir. At this time, natural gas was initially simply allowed to escape or burned to get rid of it.[32] Today, it is a fuel that provides 30% of the energy for the United States, and over one-fifth of global energy production[33]. This gas is then transported all over the world – in pipelines, in compressed natural gas tankers, and as a liquid – to be used to heat homes, in factories for heat and as a raw material, and to generate electricity the world over. Among all the fossil fuels (coal, oil, natural gas), natural gas burns the cleanest, making it popular where there is the greatest drive to reduce carbon dioxide emissions.[34]
For the purposes of electricity generation, there are two ways that natural gas is used – as fuel for a open-cycle gas turbine or in a combined-cycle gas turbine. Open-cycle gas turbines work on the Brayton Cycle[35], where air is pressurized in a compressor and mixed with natural gas before being burned. This combination of heat and pressure imparts a lot of energy into the air waiting to be released. This can only occur by moving through the turbine, whose fan blades catch the fast movement of air and spin it to generate electricity and run the compressor for the next batch of air. With lower efficiencies in the 20 to 30% range and the relatively high cost of natural gas, this type of plant is used mainly for peaking purposes. The flexibility of the fuel comes from the innovation of the combined-cycle gas turbine, as seen below.
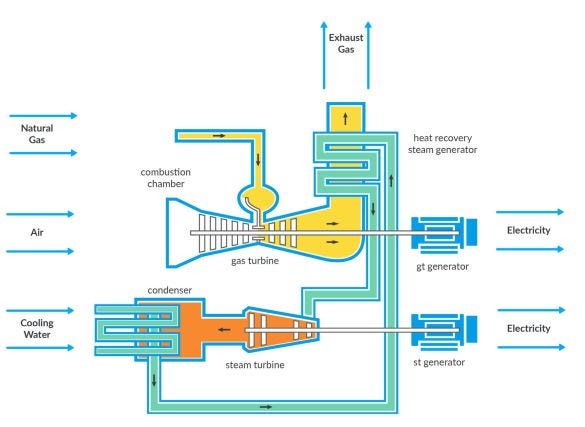
Combined-cycle gas turbines (CCGT’s) make use of the hot air coming from an open-cycle gas turbine to heat up water and drive a steam turbine. This method is called the Rankine Cycle[36], and is the same general theory used to run steam turbines in coal-fired and nuclear power plants. After the hot air spins the turbine, it is put into a heat exchanger with a series of pipes, where it is used to boil the water in the pipes. This water then provides the steam that spins the steam turbine, getting two spinning turbines out of one burn[37]. Because of this double burn, CCGT’s are very efficient, and can be used even as base-load power, if needed. In most cases, however, they will be preserved for mid-merit uses – the gas turbine can react to changes in demand much sooner than the steam turbine can, since the former has a compressor while the latter relies on heating to reach a sufficient steam pressure.
Overall, natural gas is the most flexible of all the electrical generation, and just like Queen Oil before it, is also very useful outside the world of electrical generation. It is a vital component of worldwide electricity generation, coming in second only to King Coal.
Nuclear
Policy Reaction: Scary but necessary if you’re serious about emissions.
Type: Generator (dispatchable)
Produces: Electricity
Specialty: Base load
Contribution: 9.18% of world electricity production.
If coal and oil are the King and Queen of the electrical generation world, nuclear and natural gas are the prince and princess, ready to take over their spots at the peak. Coal is an older fuel, being phased out due to carbon emissions, while oil is needed for other things such as transportation and plastics where it is somewhat impractical for electricity to replace them. Natural gas, being a cheaper feedstock that is easier to handle and use in transportation, wants to succeed Queen Oil. Nuclear is, in my opinion, the heir to King Coal’s throne as the undisputed best at base-load power.
When considered from the point of view of electrical generation, nuclear fission power plants are a lot like coal, particularly in its younger years before the different interests began causing a divergence in types and methods of construction. 96% of nuclear plants by generation that we use today are water-cooled reactors, being either light water reactors or heavy water reactors. Light Water Reactors (LWRs) use normal water, and come in two types – Pressurized Water Reactors (PWRs), which run the steam turbine by siphoning heat from the nuclear fission chamber, and Boiler Water Reactors (BWRs), which use steam produced inside the reactor core to spin the turbine. All LWRs require a high concentration the commonly-used fissile isotope, Uranium-235.[38]
Heavy Water Reactors (HWRs), by contrast, enrich the water, rather than the uranium – also an expensive process, but much less so in the long run. By using water that includes a heavier hydrogen isotope (Deuterium, which has both a proton and a neutron in the nucleus rather than just a proton), HWRs can use naturally-occurring uranium in the form of uranium dioxide (UO2), making it less expensive. Canadian-developed CANDU reactors can even use reprocessed uranium or spent nuclear fuel from LWRs.[39]
When talking about the fuel, we have to talk about uranium enrichment. LWRs and HWRs each use different fuels – the former requires expensive enrichment and the latter does not. Uranium enrichment is a long process – after mining the uranium, it is dissolved into a liquid, leached out into yellowcake, converted into uranium hexafluoride gas, centrifuged, and then processed into uranium dioxide for transit[40]. The key step in this enrichment is the centrifuge, itself an expensive piece of equipment that uses an alternating magnetic field to spin at 150,000 revolutions per minute (RPM) – ten thousand times faster than your washing machine[41]. Centrifuging the gas causes the difference in the molecular weights of the two isotopes of uranium to separate them, allowing us to increase the concentration of Uranium-235 in the final product. These enrichment facilities required to create Uranium-235 for LWRs can also create the weapons-grade uranium used for atomic bombs, while the less pure uranium used for HWRs cannot. However, the reactor will sometimes breed Plutonium-239, a very fissile isotope of plutonium that can be used in nuclear bombs.[42] There are more risks with nuclear than coal, to be sure, as fissile nuclear material is a far cry from flue ash as a weapon of mass destruction.
Once the fuel is prepared, however, the process it goes through is remarkably standard, like coal. Both LWRs and HWRs use nuclear fission. Nuclear fission is the process of bombarding the fuel atoms with neutrons until they split, releasing energy in the form of nuclear radiation and heat.[43] This heat is used to boil water, providing the steam to run a steam turbine. The common danger at this point is a nuclear chain reaction. The splitting of the fuel atoms naturally creates new neutrons, which can themselves bombard other fuel atoms and cause a chain reaction – left unchecked, the atoms split each other so fast that the heat exceeds what the reactor can take, causing a meltdown.[44] Controlling the reaction requires using different methods that can soak up the neutrons without decaying, the most popular of which are the control rods of Chernobyl fame.[45] The further into the reactor the control rods are, the more the nuclear chain reaction is suppressed, which is used to modulate the power output of the plant. These and many dangers have held back nuclear plants – the extreme effects of any failure forcing the nuclear industry to have very strict and very exhaustive processes and tight security.
Nuclear fuel can last for anywhere from three to five years, with the concentration of Uranium-235 decreasing until the fuel becomes unable to cause a nuclear chain reaction and becomes unsuitable for generation. At this point, it is moved out and either directly disposed of by hiding it away, or recycled by extracting plutonium and uranium and turned back into fresh fuel.[46] For a similarly-sized plant, coal uses huge amounts of fuel and generates up to 300,000 tonnes of ash a year, a typical 1,000 Megawatt nuclear power station will generate three cubic meters of waste per year – one of nuclear’s substantial benefits.
For all this trouble, nuclear has substantial benefits as a coal substitute. Low carbon dioxide emissions are a given – while a lot of carbon dioxide is required to build the plant, the actual generation of the plant is relatively clean. Second is a long-lasting, very reliable base-load generation source. The average life of a nuclear reactor is 40 years, with many reactors now being authorized to continue operating for 60 years – meaning plenty of time to pay off the costs of construction[47]. Finally, nuclear plants can run steam turbines at high capacity for a long time, making it an excellent chunk of power to help support the whole grid.
While everything looks like it should smell like roses, the nuclear industry has it just as hard, if not worse, than King Coal. High-profile accidents at Three Mile Island and Chernobyl, along with the tsunami that flooded the Fukushima reactor, have tarnished nuclear’s image in the eyes of politicians and citizens. Increasing concerns around safety have increased regulations and the cost of construction, preventing this form of energy from taking over. Only considering PWRs, it may be some time before nuclear truly replaces coal. The demand for safety and security from nuclear energy is high, because the potential consequences are huge.
The industry, like the coal industry did, is adapting. Molten Salt Reactors (MSRs), promising less waste and solid fuel use, are being developed as prospects in the face of nuclear waste and proliferation concerns[48]. Small Modular Reactors (SMRs), aim to comply better with regulations by building the reactors in a consistent and controllable factory environment, sticking to the approved design and building it over and over again to be shipped out into buildings.[49] Supercritical turbines are also in the works, increasing the efficiency of generation. CANDU reactors, using naturally occurring uranium fuel that doesn’t need enrichment, can significantly reduce the cost of generation.[50] These adaptations are all for the goal of making nuclear a common and competitive source of electricity – the prince is coming for King Coal’s throne.
Geothermal
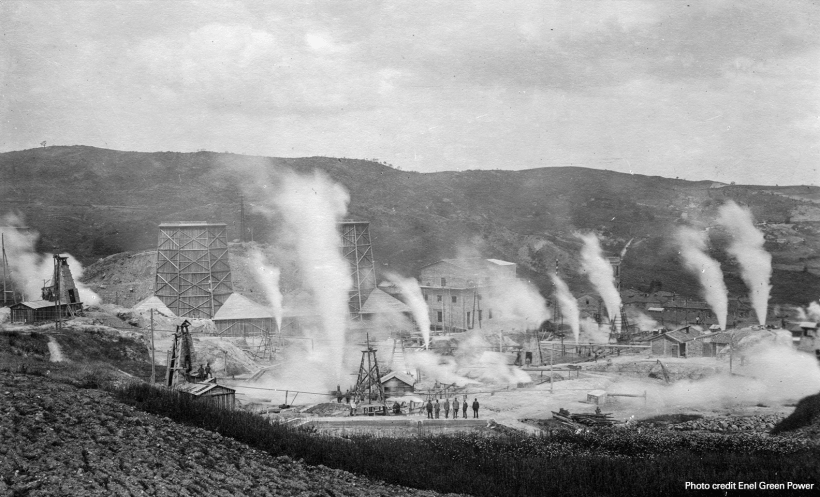
Policy Reaction: If we can find the right kind of geography for it, we’ll make more of it.
Type: Generator (dispatchable)
Produces: Electricity
Specialty: Base load
Contribution: Not reported separately by Our World in Data, likely part of Other Renewables at 0.34% of total worldwide generation.
Geothermal generation uses energy that is lurking right below our feet, out of sight and out of mind. Magma reservoirs deep underground heat a permeable layer of rock, boiling the water within and allowing the steam to escape to the surface. Just like hot springs, geothermal relies on hot water coming through permeable rock and working its way up to ground level.[51] To reach these layers, geothermal plants drill down to the permeable layer and either make use of existing natural water reservoirs to make steam, or inject water into the ground to turn into steam. From there, the steam is piped up to the surface to, as we always do, run a steam turbine. There are a few different set-ups for the turbine – dry steam, flash steam, and binary steam, all of which work best for different kinds of resources. Dry steam uses existing steam from below ground, flash steam uses existing underground reservoirs coming up because of its own heat and pressure. Both of these methods can be supplemented by water injections into the rock layer, heating it up into more steam. Binary steam uses lower temperatures than dry and flash steam, exchanging the underground heat with a working fluid with a lower boiling point than water. This allows that working fluid to spin the turbine while the water is returned below ground.[52]
Boasting low emissions and very low running costs, geothermal energy is a dispatchable source of energy that relies on only water. From this description, it seems to be on the same level as hydropower, yet hydropower generates much, much more of our electricity than geothermal does. The key limiting factor here, as in conventional reservoir hydro, is the geography. As you descend towards the Earth’s core, the ground heats up by 25 to 30 degrees Celsius for every kilometer you dig. Geothermal relies on temperatures of around 200-300 degrees Celsius. With the Al-Shaheen borehole for oil exploration reaching down over 12 kilometers, it seems we should be able to repeat this success to develop geothermal sources.[53] This, however, ignores a key constraint – the steam that reaches the surface needs to have enough pressure and temperature to run the turbines or heat the working fluid. Remember that the below-ground steam comes up under its own pressure, and a 12-kilometer long tunnel will be an impractically large tunnel to be pressurized enough to run a turbine.
As such, geothermal power is limited by access to magma, hot springs, or underground reservoirs closer to the surface. Geothermal wells are currently up to 3 km deep, with high estimates of geothermal resources shooting for 10 km deep wells, which is an extremely expensive process. Wells exceeding 4 km have digging costs in the tens of millions of US dollars – an expensive and risky investment that may not yield, because you’re never really sure what lurks underground until you dig into it.
Because of their low running costs and predictable operations due to steam, geothermal is best used as a base-load power source, consistently running in the background. Their main limitations are the availability of sites and the expenses and risks of drilling, as well as the difficulty of ramping production – remember that the majority of plants are dry steam and flash steam, which are dependent on natural forces.
Wind Turbines
Policy Reaction: Build! Build! Build!
Type: Collector (non-dispatchable)
Produces: Electricity, historically Mechanical Work
Specialty: Special, Off-Grid
Contribution: 7.32% of world electricity generation.
All the attention these days is placed on wind turbines as the large, tall steel structures that harvest energy from the movements of the wind. Electricity is generated by massive turbines, the newest ones with rotors (that’s what the span of the blades is called) over a hundred meters in diameter, with newly-installed ones reaching over 130 meters, the turbines themselves standing at 150 meters or more.[54] We forget that wind power has been with us for a long time – pumping water, grinding grain, and generating small-scale, off-grid electricity for a long time.[55] In contrast, the electricity-generating wind turbine is a relatively new format for wind turbines, and the one that is relevant for our purposes.
Commercial wind turbines are built with three blades on a horizontal axis that allow them to best generate power only when facing into the wind. Vertical-axis designs can get around this, but are less efficient overall. The actual generating turbine is mounted in a nacelle of the turbine – if you’ve seen an electric fan, the motor is in the middle of the blades.[56] In fact, a wind turbine is in many ways an electric fan operating in reverse, catching wind to generate electricity, rather than using electricity to generate wind. These turbines have gearboxes and other methods of controlling the movement for maximum generation efficiency in different wind speeds, but they also have one other feature – the ability to disconnect. Excessively high wind speeds will damage the turbine’s internals, breaking the insides and preventing the turbine from generating electricity. The turbine prevents this by simply shutting off under too-high winds, meaning that generation doesn’t occur at speeds exceeding design capacity.[57]
The key problems with wind turbines all stem from the delicate balance of bigger turbines chasing larger and variable winds. Bigger turbines are more efficient, since larger rotors can catch more surface area and deliver more energy to the turbine. However, the blades and turbines need to be made of stronger materials and designs to keep up with stronger winds, increasing the weight and making them difficult to build and transport.[58] This would be fine, if wind was consistent, but it is highly variable. While the average wind speed may be well within the design parameters, the wind turbine has no control over the wind simply stopping in a dead calm, or going so fast that the turbine would be damaged. In those situations, the turbine is disconnected from generation and allowed to spin freely.[59] All these factors result in a very variable generation that usually averages out on the grid scale but needs to be backed up by firm capacity for a truly reliable grid.
Financially, wind turbines share the same pattern as nuclear, geothermal, and solar panels – heavy capital investment up front, and low to no fuel costs going forward. This has led to generation from these sources being able to undercut other sources of electricity – keep this in mind for the next part of the Guide.
Solar Panels
Policy Reaction: Even the people should get these on their roofs!
Type: Collector (non-dispatchable)
Produces: Electricity
Specialty: Special, Off-Grid
Contribution: 4.57% of world electricity generation.
Solar energy, as a whole, is probably the oldest form of energy. Photosynthesis, drying meat, fish, and fruit, growing food – all of these things have always relied on solar energy. The advent of solar concentrators and photovoltaic solar generation is simply the most recent innovation in the solar power universe.
The vast majority of solar power generation is photovoltaic – generating power directly through the difference in electron excitement when the solar panel is hit by the sun. The photovoltaic effect[60] involves sunlight causing the electrons in a material to be excited and be ejected from a material, taking their charge with them. Solar panels use semiconductors[61] like silicon or other metal alloys in thin wafers or films to “catch” these electrons and take their energy. They eventually return to the other side via diffusion, as the balance of electrons in the cell needs to be restored. This generates direct current (DC), which needs to be converted to alternating current (AC) to be used in the grid.
For a relatable example, think of a beach vacation. As winter approaches, and people living in colder climates dream of the hot sand and sun (excitation), there is a mass movement of people (electrons) from colder areas to warmer areas, where they spend a lot of money (electron charge) on accommodations, luxuries, and other stuff, before going back home when it’s less unbearably cold (diffusion).
Photovoltaic panels used in utility-scale solar farms can be fixed or come with small motors that track the sun. Inverters and transformers sit among the sea of silicon solar panels, converting the DC generated by the panels to AC before sending it out to the grid at the right voltage and frequency.[62] Solar panels, in contrast to the other forms of electricity covered so far, are simple in concept – point it at the sun and let the electricity come in. Though, as always, there are hitches.
There are two large hitches that prevent solar from simply taking over the world – land area and unpredictability. Solar farms, by their nature, need to be spread out to catch as much sun as possible, in order to generate electricity. Our World in Data estimates that photovoltaic solar farms require 19 square meters per MWh, compared to gas and nuclear sitting down at 1 square meter per MWh or less[63]. This is not helped by the unpredictability of solar – you can lose a lot of output when a cloud floats between the sun and your facility, and obviously, solar panels provide no generation at night – neither of which you have any control over.
Overall, photovoltaic solar is a great energy source if you have a load that matches well with solar irradiation. One such load is air conditioning and cooling – demand for these services is greatest in the middle of the day when solar should also be at its peak. Not having such a load creates the notorious duck curve – an oversupply of solar when it is collected but not needed, and an undersupply for the grid when it is needed but not collected.[64] While this surplus could be used at a grid scale to enable pumped hydroelectricity, that isn’t always an option – leading to battery storage.
Battery Storage
Policy Reaction: Our savior from duck curves, the vanquisher of intermittency, and a path to a Net Zero future!
Type: Pure Storage (does not generate)
Produces: Electrical Storage
Specialty: Special
Battery storage is the new kid on the energy block, and though we’ve been using batteries in smaller applications for a long time, the use of them at grid scale is certainly novel. Grid-scale battery storage systems generally prefer to use lithium-ion or lithium-iron-phosphate batteries for their large high energy efficiency, density, and cycle count.[65] However, relying on lithium makes them sensitive to the prices of this metal, and so other opportunities are sought out. Flow batteries and metal-free alternatives are being researched, but aren’t quite ready for prime time yet.
The key difference between battery storage as a matter of generation and the battery storage already in use is the scale. We already use batteries for hybrid/off-grid applications to store energy from renewables, or to stabilize smaller local microgrids (say, for a village) that are isolated from the wider grid and need their own backup.[66] Grid-scale battery storage, however, is much larger and more concentrated than these uses, storing up to thousands of Megawatt-hours (MWh) of ready power.[67] Let’s take the second-largest one as an example – the Vistra Moss Landing Battery, in California. Boasting a 750 Megawatt discharge capacity and 3,000 Megawatt hours, the battery can discharge, in an emergency, 4 hours of power at full blast, in theory.[68] The California grid as a whole, in 2018, generated and used 193,075 Gigawatt hours (GWh, each one being 1,000 MWh), plus 19,828 GWh from rooftop solar, for a total of 212,903 GWh, or 212,903,000 MWh – divided by the number of hours in the year (8,760), that is 24,304 MWh per hour, on average. At a rated discharge of 750 MWh, you would need about 33 of the Vistra Moss Landing Battery to power California for 4 hours. Is that good or bad? Perhaps this level of capacity is sufficient if you’re just supporting the grid and responding to peak loads, providing voltage and operating reserves where they are needed, but it might be questionable for storage over a longer period of time?
Though beyond the scope of this part of the Guide, I invite you to do your own math and come to your own conclusions.
Conclusion
After this whirlwind, top-level tour of all the methods we use to generate electricity, next on the docket is a short part, where I will talk about capacity factor, grid transmission, and reliability – still falling under the umbrella of generation, but now with the knowledge of how different sources like to contribute to the grid. We can finally, finally talk about some of the hot, top-level topics that reach the layman – grid stability, spinning reserve, voltage and frequency maintenance, and spot markets! Look forward to it, as I describe the process of how we settle who generates what.
Support the Guide
You can support the Guide by sharing this post. If you want to know more, subscribe to The Professional Amateur so you don’t miss the next part!
If you have a question, feel free to comment or message me about it - I love to talk electricity!
If you really liked the Guide, I also have a Ko-Fi page where you can buy me a coffee to help get this Guide out faster. The Guide is a labor of love, but it is still a labor, so a committed show of support from you enjoyers out there would be greatly appreciated. Leave your Substack handle as a message to me, and I’ll be including you in the sponsor roll on the next part - members get their own section!
[1] https://ourworldindata.org/electricity-mix
[2] https://en.wikipedia.org/wiki/Treadwheel_crane
[3] https://en.wikipedia.org/wiki/Horse_mill
[4] https://en.wikipedia.org/wiki/Biomass_(energy)
[5] https://en.wikipedia.org/wiki/Dry_dung_fuel
[6] https://www.ncbi.nlm.nih.gov/pmc/articles/PMC1262769/
[7] https://en.wikipedia.org/wiki/Log_driving
[8] https://en.wikipedia.org/wiki/Water_wheel
[9] https://en.wikipedia.org/wiki/Run-of-the-river_hydroelectricity
[10] https://www.energy.gov/eere/water/types-hydropower-plants
[11] https://www.hydroreview.com/technology-and-equipment/generators-and-electrical-components/hydroelectric-plants-have-fastest-start-up-time-of-u-s-electric-generators/
[12] https://en.wikipedia.org/wiki/Dam_failure
[13] https://en.wikipedia.org/wiki/Hydroelectricity
[14] https://www.benarnews.org/english/news/philippine/dam-protest-02232023140543.html
[15] https://en.wikipedia.org/wiki/Hydroelectricity#Ecosystem_damage_and_loss_of_land
[16] https://www.energy.gov/eere/water/pumped-storage-hydropower
[17] http://history.alberta.ca/energyheritage/coal/early-coal-history-to-1900/the-steam-engine-the-industrial-revolution-and-coal.aspx
[18] https://energyeducation.ca/encyclopedia/Coal_types
[19] https://en.wikipedia.org/wiki/Coal-fired_power_station#Fuel_processing
[20] https://en.wikipedia.org/wiki/Coal-fired_power_station#Fuel_processing
[21] https://en.wikipedia.org/wiki/Circulating_fluidized_bed
[22] https://en.wikipedia.org/wiki/Integrated_gasification_combined_cycle
[23] https://en.wikipedia.org/wiki/Integrated_gasification_combined_cycle
[24] https://www.gem.wiki/Coal_power_technologies
[25] https://www.gevernova.com/steam-power/coal-power-plant/usc-ausc
[27] https://www.encyclopedia.com/history/encyclopedias-almanacs-transcripts-and-maps/black-gold
[28] https://www.investopedia.com/articles/investing/102314/understanding-benchmark-oils-brent-blend-wti-and-dubai.asp
[29] https://www.mckinseyenergyinsights.com/resources/refinery-reference-desk/residual-fuel-oil/
[30] https://www.eia.gov/tools/glossary/index.php?id=distillate
[31] https://www.eia.gov/energyexplained/use-of-energy/transportation.php
[32] https://en.wikipedia.org/wiki/Natural_gas
[33] https://afdc.energy.gov/fuels/natural_gas_basics.html
[34] https://energyeducation.ca/encyclopedia/Natural_gas
[35] https://en.wikipedia.org/wiki/Brayton_cycle
[36] https://en.wikipedia.org/wiki/Rankine_cycle
[37] https://engie.com.au/home/about-engie/education/how-does-gas-fired-power-work
[38] https://www.iaea.org/topics/water-cooled-reactors
[39] https://www.iaea.org/topics/water-cooled-reactors
[40] https://www.iaea.org/newscenter/news/what-is-uranium
[41] https://en.wikipedia.org/wiki/Zippe-type_centrifuge
[42] https://en.wikipedia.org/wiki/Pressurized_heavy-water_reactor
[43] https://www.iaea.org/newscenter/news/what-is-nuclear-energy-the-science-of-nuclear-power
[44] https://en.wikipedia.org/wiki/Nuclear_chain_reaction
[45] https://en.wikipedia.org/wiki/Control_rod
[46] https://world-nuclear.org/nuclear-essentials/what-is-nuclear-waste-and-what-do-we-do-with-it.aspx
[47] https://www.iaea.org/topics/water-cooled-reactors
[48] https://www.iaea.org/topics/molten-salt-reactors
[49] https://www.iaea.org/topics/small-modular-reactors
[50] https://energyeducation.ca/encyclopedia/CANDU_reactor
[51] https://www.fujielectric.com/products/energy/geothermal_power_generation/index.html
[52] https://www.nrel.gov/research/re-geo-elec-production.html
[53] https://en.wikipedia.org/wiki/Al_Shaheen_Oil_Field#Records
[54] https://www.energy.gov/eere/articles/wind-turbines-bigger-better
[55] https://en.wikipedia.org/wiki/History_of_wind_power
[56] https://en.wikipedia.org/wiki/Wind_turbine#Horizontal_axis
[57] https://en.wikipedia.org/wiki/Wind_power#Turbine_design
[58] https://www.energy.gov/eere/articles/wind-turbines-bigger-better
[59] https://www.wind-energy-the-facts.org/understanding-variable-output-characteristics-of-wind-power-variability-and-predictability.html
[60] https://en.wikipedia.org/wiki/Photovoltaic_effect
[61] https://en.wikipedia.org/wiki/Solar_cell#Materials
[62] https://en.wikipedia.org/wiki/Photovoltaic_power_station#Technology
[63] https://ourworldindata.org/land-use-per-energy-source
[64] https://www.synergy.net.au/Blog/2021/10/Everything-you-need-to-know-about-the-Duck-Curve
[65] https://www.azom.com/article.aspx?ArticleID=22683
[66] https://www.sintef.no/en/expertise/sintef-energy-research/integration-of-batteries-in-the-electricity-grid/
[67] https://en.wikipedia.org/wiki/Battery_storage_power_station
[68] https://en.wikipedia.org/wiki/Moss_Landing_Power_Plant#Vistra_500_kV
Very in-depth exploration into all things energy production whether electrical, mechanical, heat or transport. Its clear how much work went into this and its well worth a read! I found it especially useful to review the policy attitudes towards these methods.
As Argo states:
"If coal and oil are the King and Queen of the electrical generation world, nuclear and natural gas are the prince and princess, ready to take over their spots at the peak."
The world is changing, and many of the methods included in this piece will play a huge part in this transition.
Nice guide. However, there is a glaring error in your estimate of the area required to generate 1 megawatt via solar PV. First, the energy coming from the Sun directly overhead on a clear day can be approximated by 1 kilowatt per square meter. The conversion efficiency of polycrystalline silicon is about 10%, meaning that 10 square meters are required to generate 1 kilowatt. Thus 10,000 square meters would be required if all of the area is covered. Let's use a more realistic 50% coverage to calculate 20,000 square meters of area is required. That works out to a square 141 meters on a side, instead of the 19 meters on a side shown in your text.